Current Research
Harnessing Redox-Active Ligands
Non-innocent or redox-active ligands have been long known to complicate the assignment of oxidation states in transition metal complexes and to participate in the redox behavior of these complexes. Our research program aims to harness the properties of these ligand systems to effect new stoichiometric and catalytic reaction chemistry. Multi-electron transformations can be addressed through the use of metal and ligand valence changes. We are currently applying this concept to the fundamental chemical challenges of some of the most important problems facing the world today:
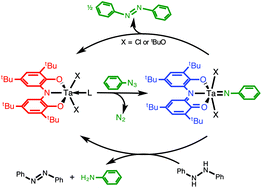
Small Molecule Activation
The efficient reduction of small molecules, such as N2, CO2, and related small molecules substrates, requires catalysts capable of delivering multiple electrons and multiple protons in a concerted fashion. Redox-active ligands provide an attractive way to tackle these challenges. The electronic structure of redox-active ligands allows them to deliver multiple reducing equivalents with minimal reorganizational barriers; furthermore, as the ligand delivers reducing equivalents (electrons), it becomes more acidic and can readily deliver protons.
In the Heyduk group, we have shown that ligand-based multi-electron redox reactivity is readily achieved from many of our metal complex systems. We are designing metal complexes with multiple redox active ligands to catalyze the two, four, and six-electron reduction of various organic substrates.
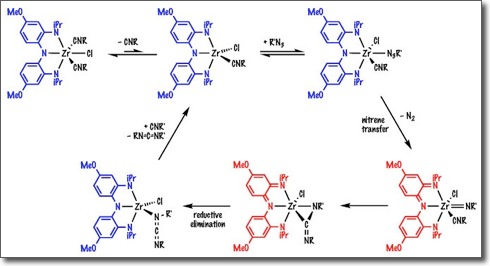
Group- and Atom-Transfer Reactivity
Mid- and late-transition metal imido and oxo complexes often display atom and group transfer reactivity because the metal centers can readily change oxidation state. In the case of imido and oxo complexes of the early transition metals, the lack of metal-based redox reactivity typically prevents their use as atom and group transfer reagents; however, a high degree of polarization in the metal-ligand multiple bond often leads to other reactivities such as C–H bond activation.
Our group seeks to combine these disparate reactivity modes by utilizing redox-active ligands to support early transition metal imido and oxo complexes. By tuning the electronic and steric properties of the complex both C–H bond activation and group-transfer reactivity should be accessible providing a rational design platform for catalytic C–H bond amination and oxidation.
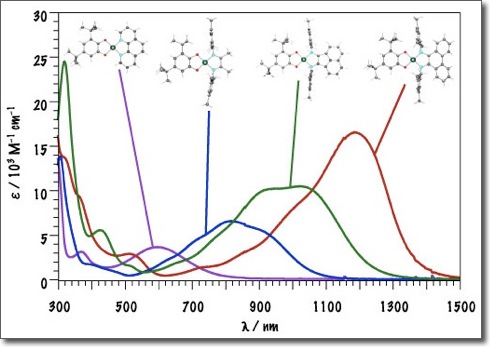
Artificial Photosynthesis
Artificial photosynthesis is our attempt to capture the kinetic energy of the Sun and store it in chemical bonds. In Nature, this is routinely done by green plants and photosynthetic bacteria. The Sun’s light is captured the energy is stored by converting water and carbon dioxide to oxygen and sugar. Artificial photosynthesis requires four steps:
We are designing new molecules to tackle each of the above steps, with the idea that they can then be assembled in a modular fashion to form macromolecular or supramolecular assemblies that act as a molecular machines for artificial photosynthesis.